Achieving higher amplification efficiencies of RNA
In situ sequencing (ISS) ST methods have allowed targeted RNA to be analyzed in preserved cells and tissue sections. For ISS approaches that employ a gap-filling padlock-based approach, overextension of the padlock probe by DNA polymerases with strand displacement activity may occur, which prevents ligation and decreases amplification efficiency. Researchers faced the challenge of ensuring optimum amplification efficiency for gap filling approaches in the study by Chen et al., in 2018. The researchers identified a DNA polymerase without strand displacement activity — Phusion DNA polymerase — that produced much less overextension of the padlock probes, resulting in more efficient gap-filling. The developed method was the Barcode in situ targeted sequencing (BaristaSeq) technique1. The study achieved a five-fold increase in amplification efficiency compared to the traditional enzyme employed in the conventional gap-filling approach — because of a lack of strand displacement activity1.

Avoiding efficiency-limiting steps
Another challenge for ISS protocols is ensuring efficiency in the RNA-to-complementary DNA (cDNA) conversion step by reverse transcription (RT), which is considered inefficient and variable. One way to solve this issue is to eliminate the RT step. The method published in Science in 2018, named Spatially Resolved Transcript Amplicon Readout Mapping (STARmap), did precisely that —replacing the RT step by employing a pair of primer and padlock probes that hybridize to the same RNA followed by enzymatic amplification to produce a DNA nanoball (amplicon). Consequently, errors in data signal generation are also avoided as the probes only bind when perfect matches occur and are consequently stripped away following imaging2. Hydrogel-tissue chemistry employed in the technique allowed the DNA amplicons to be embedded into the 3D tissue scaffold. STARmap has avoided the efficiency hurdle of cDNA conversion and achieved highly accurate and efficient simultaneous mapping of 160 to 1020 genes in mouse brain sections. Further, replacing the RT step in the STARmap method greatly improved specificity (by reducing the non-specific hybridization of single probes) and the efficiency of transcript capture.
Automation for clinical laboratories
To reap the benefits of ST in high-throughput patient tumour screening, ST protocols must be successfully integrated into clinical diagnostic workflows. The challenge in doing this is automating protocols to reduce the time taken to perform protocols in busy clinical laboratories. The research article by Maino et al., tackles this challenge by describing an automated ST method that can be performed in clinical diagnostic laboratories. Here, the ISS technique was adapted to an automated microfluidic system. The developed system involved a programmable system to deliver reagents in a temperature- and flow-controlled manner, which supported ISS protocols comprising of hybridization and ligation of gene-specific padlock probes and rolling circle amplification of probes and barcode sequencing using sequencing-by-ligation (SBL) chemistry. Although the automated technique demonstrated the same performance as the manual ISS protocols, the automation provided supplementary benefits such as reduced hands-on time (3h 12 mins compared to 4h 38 mins) and increased assay speed (10h compared to 18h 37 mins)3. Certain assay parameters of the automated method were not as ideal as the manual technique, such as the higher consumption of enzymes (1.5x higher than the manual ISS protocol), the benefits of time-saving and lower consumption of oligonucleotide (2.5x reduction) make the automated ISS technique very useful for routine clinical analysis3.
Improving the accuracy of imaging the transcriptome in situ

Another challenge of imaging the transcriptome in situ is obtaining high accuracy. Accuracy can be reduced by optical crowding from other transcripts— the combined effect of reaching the limits of optical resolution and the high density of transcripts in the cells. The sequential fluorescence ISH (seqFISH) technique identifies mRNA directly by performing multiple rounds of hybridization, imaging, and probe stripping. A further development of the seqFISH approach is called seqFISH+, which has overcome the optical crowding problem by expanding the barcode base palette to localize the mRNA. Whilst seqFISH uses 4-5 colors and sequential hybridization, seqFISH+ employs 60 pseudocolors divided into three fluorescence channels (Alexa 488, Cy3b and Alexa 647). The seqFISH+ protocol has imaged mRNAs for 10,000 genes in single cells in situ from different areas of the mouse brain (cortex, sub-ventricular zone, and olfactory bulb)4, therefore increasing the accuracy and resolution of imaging the transcriptome in situ.
Reducing non-specific autofluorescence signals from tissues
A common issue with ST methods is the high levels of background signals arising from autofluorescence in tissue samples, which can complicate signal acquisition and analysis. Imaging of human brain samples, particularly those of aging brains, is hampered by strong autofluorescence in multiple channels caused by lipid-containing residues of lipofuscin. This problem is compounded by the fact that human brains are much larger than those from other animal models often used to characterize ST methods. To reduce the problem of autofluorescence, ST methods have relied on additional computational methods, which are time-consuming. The Hybridization-based ISS(HybISS) ST method process employs a simple autofluorescence quenching step that circumvents the need for additional microscopic strategies or computational tools. This autofluorescence quenching step only adds five minutes to the protocol, and one-time treatment lasts well for image cycles5. The HybISS, together with the autofluorescence method, reduces non-specific background signals in different channels and achieves a clear separation of signals. This has been critical in distinguishing gene transcripts across entire human brain sections.
Deciphering heterogeneity in tumoral tissues to achieve better treatment
Studying heterogeneity in different cancers is essential as the subpopulations may give rise to distinct genotypes and phenotypes, some of which have malignant features, such as the ability to metastasize. In clinical diagnostics, deciphering heterogeneity in tumors is essential for deciding on the most appropriate therapeutic option. Few studies have examined the transcriptional intra-tumoral heterogeneity. Therefore, studies in this area are vitally needed. A digital spatial profiling technique named GeoMx can define a specific region of interest in tissue. It quantifies RNA abundance by counting unique indexing oligos assigned to each target of interest. The GeoMx DSP technique has become commercially available and particularly important for spatial transcriptomic profiling of highly heterogeneous tumoral tissues. In a recent study by Kim et al., and in collaboration with NanoString Technologies, researchers used the GeoMx approach to examine the microheterogeneity of the glioblastoma microenvironment. The study assessed glioblastoma tissue using samples sequenced via NGS Illumina technology by randomly selecting regions of interest (ROI) from each tissue. The technique has allowed the quantification of molecular heterogeneity of the glioblastoma microenvironment and revealed region-specific microenvironment gene expression profiles. Additionally, the GeoMx technique was ideal for studying archived tissue as it required minimal amounts of tissue to generate robust spatially transcriptional profiled datasets6. This research is a step forward in assessing the suitability of treatments for the heterogeneous glioblastoma microenvironment.

Analyzing human samples with poor RNA quality
Human samples can often have poor RNA quality. This is the case for gut-associated tissue, which contains digestive enzymes that can rapidly degrade RNA, which can produce misleading transcriptomic data. Researchers have addressed this issue by modifying a commercially available spatial transcriptomics platform, 10X Genomics’ Visium technology. In the past, one of the criteria for using Visium technology has been the integrity of RNA, defined by the RNA Integrity Number (RIN). The standard Visium protocol recommends having a RIN ≥ 7. The modified protocol, developed by Mirzazadeh et al., is RNA-Rescue Spatial Transcriptomics (RRST), with three main modifications. The protocol included formalin fixation instead of methanol and an additional baking step to prevent detachment and reinforce tissue section adhesion. The last modification was removing the crosslink-reversal step, further preventing RNA degradation. Employing the RRST method achieved higher detection rates for different regions of tissues with moderate to low RNA than the standard Visium protocol7.
References
1. Chen, X., et al. Efficient in situ barcode sequencing using padlock probe-based BaristaSeq. Nucleic Acids Res46, (2018).
2. Wang, X. et al. Three-dimensional intact-tissue sequencing of single-cell transcriptional states. Science (1979)361, (2018).
3. Maïno, N. et al. A microfluidic platform towards automated multiplexed in situ sequencing. Sci Rep9, (2019).
4. Eng, C. H. L. et al. Transcriptome-scale super-resolved imaging in tissues by RNA seqFISH+. Nature568, (2019).
5. Gyllborg, D. et al. Hybridization-based in situ sequencing (HybISS) for spatially resolved transcriptomics in human and mouse brain tissue. Nucleic Acids Res48, (2020).
6. Kim, Y. et al. Highly Multiplexed Spatially Resolved Proteomic and Transcriptional Profiling of the Glioblastoma Microenvironment Using Archived Formalin-Fixed Paraffin-Embedded Specimens. Mod Pathol36, (2023).
7. Mirzazadeh, R. et al. Spatially resolved transcriptomic profiling of degraded and challenging fresh frozen samples. Nat Commun14, (2023).
Supplier
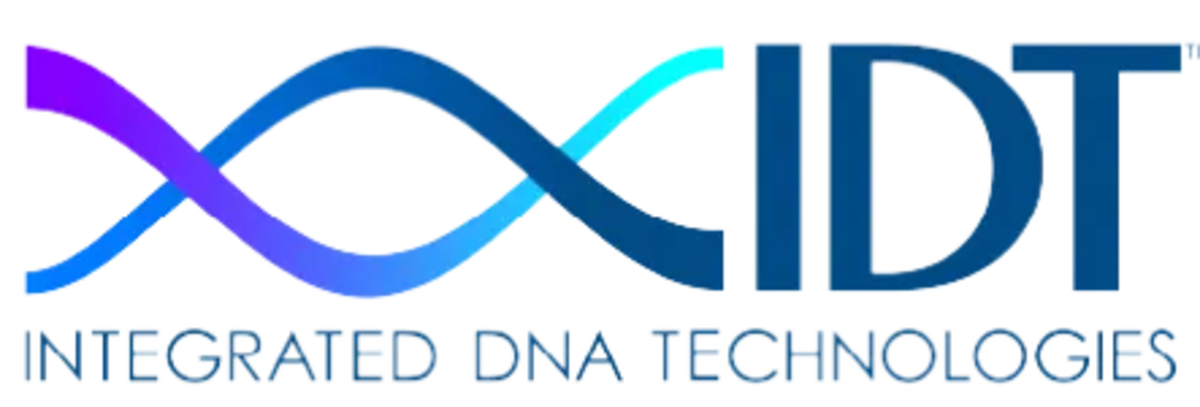
IDT - Integrated DNA Technologies
With over 30 years experience as a manufacturer, IDT offers innovative tools for NGS, CRISPR, qPCR and PCR. IDT offers superior quality DNA and RNA oligos, genes, gene fragments, Cas nucleases and more, with fast turnaround times!
About IDT Shop for IDT products
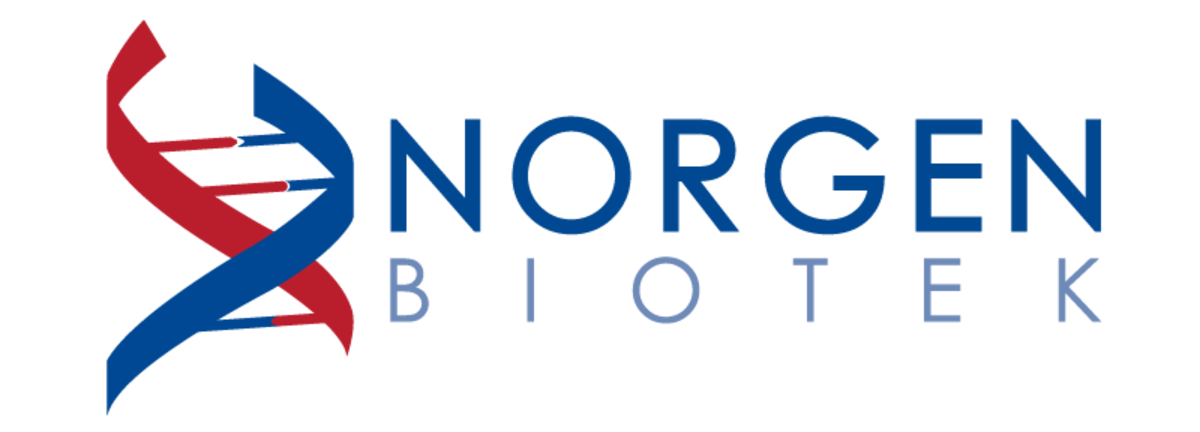
Norgen Biotek
In addition to the topselling Total RNA Purification Kit line, Norgen also offers collection devices for whole food (cf-RNA/DNA) urine, saliva and more.
About Norgen Biotek Shop for Norgen Biotek products
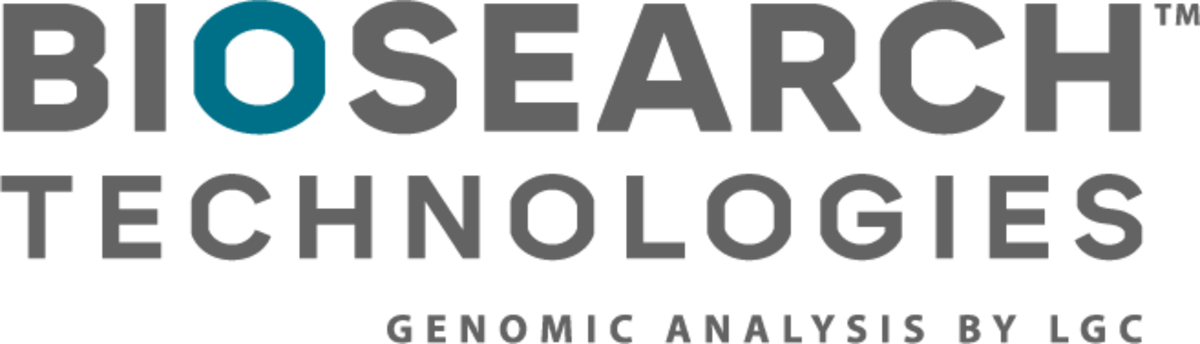
LGC Biosearch Technologies
Biosearch Technologies™ provides products and services for genomic analysis that support mission critical applications for global customers in agrigenomics and human healthcare. In addition, they provide the well-known Stellaris® RNA FISH probes.
About LGC Biosearch Technologies Shop for LGC Biosearch Technologies