What is CRISPR genome editing?
CRISPR (clustered, regularly interspaced short palindromic repeats) is a gene editing technology that silences genes at a DNA level. CRISPR can be described as a pair of molecular scissors that cuts DNA of any origin at a specific site and then modifies or deletes DNA sequences. CRISPR is the most popular gene editing tool used today and is used across a range of disciplines. The inventors of CRISPR technology have been recognized for their contribution to science and, in 2011, were awarded the Nobel Prize in Chemistry. In contrast to gene editing with RNA interference, CRISPR technology offers permanent and complete gene knockout.
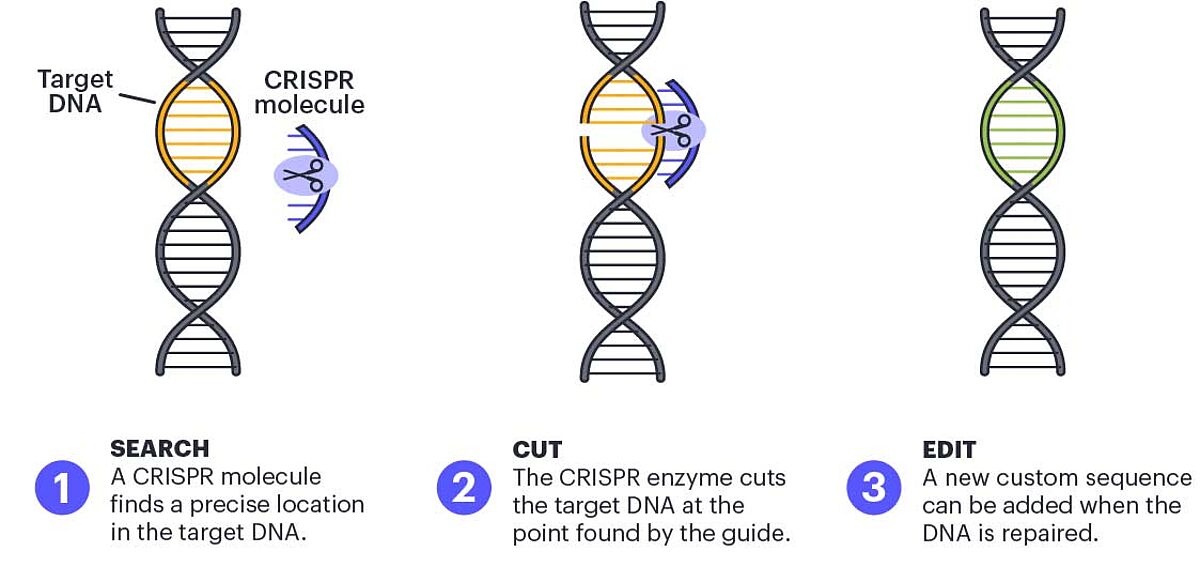
CRISPR technology relies on the nuclease activity of the bacterial endocuclease Cas – most often Cas9 – which is targeted to a specific DNA sequence using a guide RNA. Cas cuts both strands of the DNA double helix, creating what is referred to as a "double-stranded break". These breaks are repaired by the host cells' repair machinery by non-homologous end-joining (NHEJ) or by homology-directed recombination (HDR). However, when incorrectly repaired, double-stranded breaks give rise to mutations, genomic instability and cell death.
Technological advances in CRISPR
While the classical CRISPR-Cas9 genome editing technology is used in many research labs worldwide, the technology is evolving to become better and more precise. Recent progresses in CRISPR genome editing include:
1. Recent advances in CRISPR technology employ a Cas9 nickase and a modified guide RNA. Double-stranded DNA breaks are bypassed as single-stranded breaks, or no breaks are made by a Cas9 nickase fused to reverse transcriptase. These technological advances are known as prime editing.
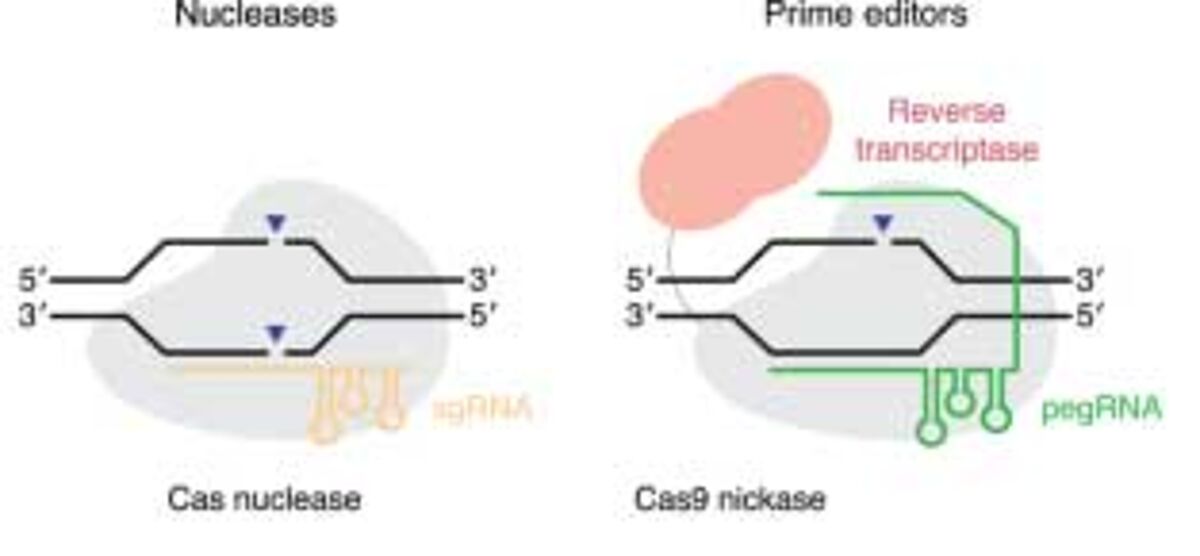
2. A recently described technological advance in CRISPR gene editing that is similar to prime editing is called PASTE for Programmable Addition via Site-specific Targeting Elements. The similarity between prime editing and PASTE is the use of a CRISPR-Cas9 nickase. However, the difference is that Cas9 is fused to two enzymes — reverse transcriptase and serine integrase, to allow the integration of large fragments of DNA. The study, published in Nature Biotechnology, shows DNA integration of up to ~36 kb into several types of human cells and different locations in the genome. Additionally, like prime editing, PASTE shows no off-target activity — so no unintended genomic changes occur at sites other than the target site.
3. CRISPR technology is restricted by its reliance on recognizing target sequences that have protospacer adjacent motifs (PAMs). Researchers have now developed a variant of Cas9 called SpRY that does not require recognition of the PAM site. The team of researchers targeted more than 130 DNA sequences using SpRY and gRNA and found that the method could cleave DNA at any sequence programmed by the gRNA, regardless of the presence of PAM sequences. Therefore this approach can now extend the target sites of CRISPR.
Alternative Cas nucleases
Cas9 is the most common form of the enzyme with nuclease activity. However, recent CRISPR discoveries mean that there are a number of Cas enzymes that can be used as alternatives to Cas9 (Table 1).
Protein | Main use/characteristics | Year/s |
---|---|---|
Cas12 | Cas12a is smaller and simpler than Cas9; Cas12b, i.a., for plant genome engineering1,2 | |
Cas13 | for RNA editing3 | |
Cas34,5 | Creates a single-stranded wide gap6 | 2019 |
CasMINI | About twice as compact as the more commonly used Cas9 and Cas12a7,8 | 2021 |
SuperFi-Cas9 | More accurate without a reduction in the cleavage rate of on-target DNA9,10 | 2022 |
Cas7-11 | Better suited for therapeutic RNA editing than Cas1311 | 2022 |
Chromosome-templated DNA repair | Such a method is only applicable to organisms whose matching chromosome has the desired gene/s. Cas9-derived nickases (D10A and/or H840A ) that nick rather than cleave target DNA) mediated homologous chromosome-templated repair method was demonstrated to be more effective than Cas9 and to cause fewer off-target edits12,13 | 2022 |
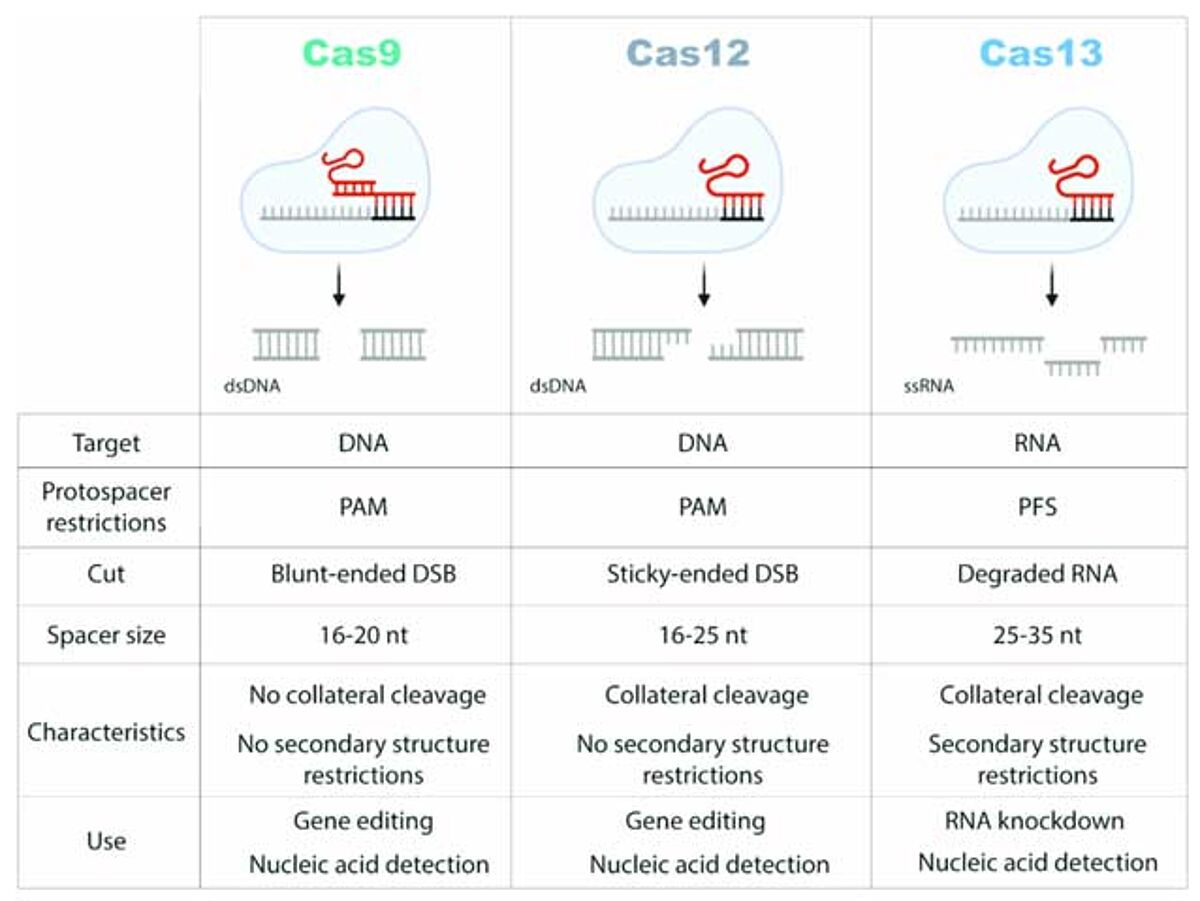
Comparison of the properties of CRISPR/Cas9, /Cas12 and/Cas13 systems. PAM: protospacer adjacent motif; PFS: protospacer flanking site; DSB: double-strand break.15
Novel applications of CRISPR genome editing
CRISPR technology is used in several areas of scientific research, medicine and agriculture. For medicine, CRISPR applications used for somatic cells have no risk of being passed on to future generations. CRISPR technologies are currently being evaluated in clinical trials for several diseases, including blood disorders, infectious diseases and cancer.
In November 2022, CRISPR gene editing was used to alter patients' own immune cells (T cells) to recognize disease-causing proteins expressed in solid tumours. The trial published in Nature enrolled 16 patients with solid tumours. Even though the success rate was limited to five patients with no disease progression, the clinical trial (NCT03970382) provides evidence for using the CRISPR technique in humans as a replacement for personalized cell therapy.
CRISPR editing applications have been tested in agriculture and farming to alter and increase the nutritional content of food. For example tomatoes have been edited with CRISPR. (Blog) In a recent study, researchers knocked out the biosynthetic enzymes responsible for converting the vitamin D precursor, 7-Dehydrocholesterol (7-DHC), to cholesterol. This produced elevated levels of the vitamin D precursor. By treating with UVB light, the vitamin D precursor can produce vitamin D. The study shows that CRISPR has important implications for increasing food's nutritional value at a time when many people worldwide suffer from vitamin D insufficiency.
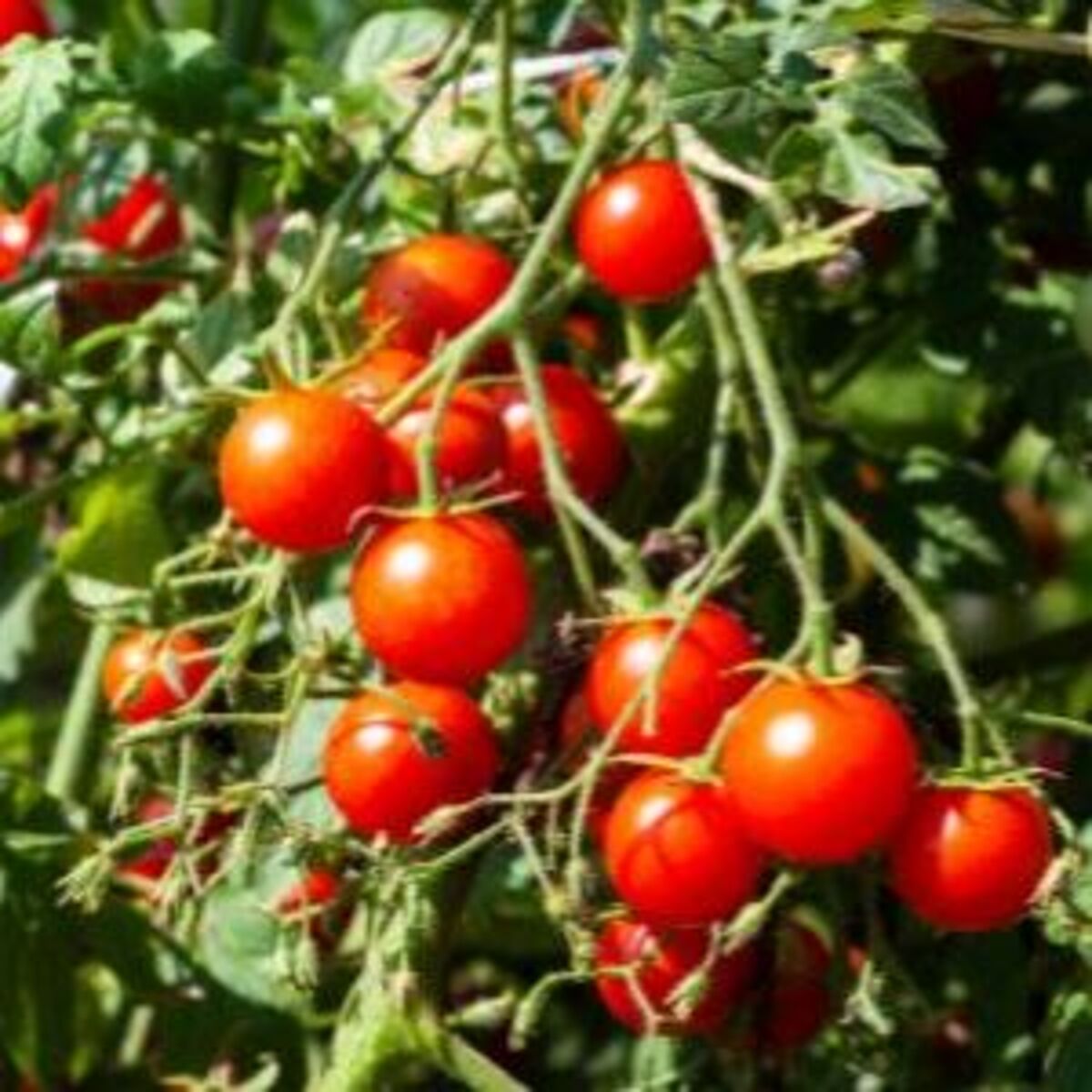
CRISPR technology is also providing solutions for infectious diseases, such as COVID-19/SARS-CoV-2. The main challenge of providing protection against COVID-19 is keeping up with the virus's ability to mutate, i.e., change its structure and evade the immune response. A recent study depicts how CRISPR, delivered using lipid nanoparticles, can effectively inhibit diverse coronavirus structures whilst avoiding any off-target effects.
The number of CRISPR applications continues to rise. Initially discovered in E. coli, the CRISPR system has now been identified in bacteriophages, presumably acquired from the bacterial host. A new study by Doudna and the team highlightthe importance of CRISPR-Cas enzymes from bacteriophages and their potential usefulness in editing genomes of plant and human cells.
IDT
Integrated DNA Technologies (IDT) is your advocate for the genomics age. They produce tools for NGS, CRISPR, qPCR and PCR. Their products include DNA/RNA oligos, genes and gene fragments. For more than 30 years, IDT's innovative tools and solutions for genomics applications have been driving advances that inspire scientists to dream big and achieve their next breakthroughs.
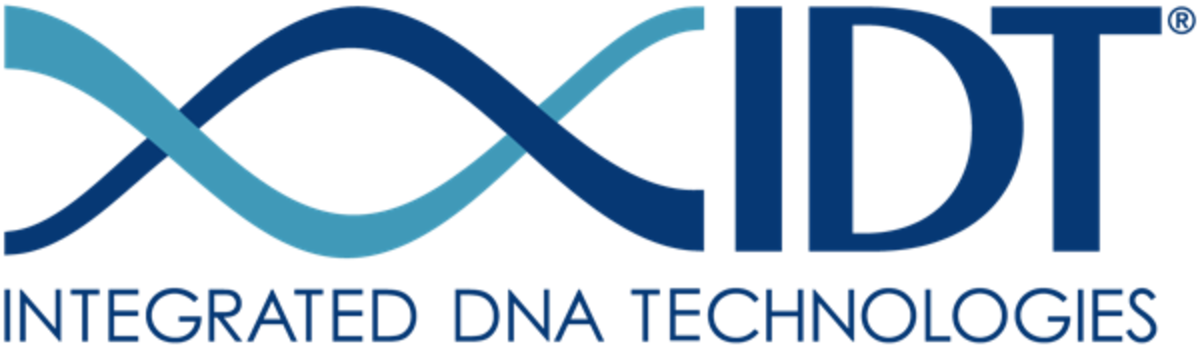
References
- Phys.org. Researchers establish new viable CRISPR-Cas12b system for plant genome engineering. Published April 6, 2020. Accessed December 22, 2022. phys.org/news/2020-03-viable-crispr-cas12b-genome.html
- Ming M, Ren Q, Pan C, et al. CRISPR–Cas12b enables efficient plant genome engineering. Nat Plants. 2020;6(3). doi:10.1038/s41477-020-0614-6
- Cox D, Gootenberg JS, Jonathan S, et al. RNA editing with CRISPR-Cas13. Science (1979). 2017;358(6366). doi:10.1126/science.aaq0180
- Dolan AE, Hou Z, Xiao Y, et al. Introducing a Spectrum of Long-Range Genomic Deletions in Human Embryonic Stem Cells Using Type I CRISPR-Cas. Mol Cell. 2019;74(5). doi:10.1016/j.molcel.2019.03.014
- Cornell Chronicle. CRISPR-Cas3 innovation holds promise for disease cures, advancing science. Published October 2021. Accessed December 22, 2022. news.cornell.edu/stories/2019/04/crispr-cas3-innovation-holds-promise-disease-cures-advancing-science
- Liu Z, Dong H, Cui Y, Cong L, Zhang D. Application of different types of CRISPR/Cas-based systems in bacteria. Microb Cell Fact. 2020;19(1). doi:10.1186/s12934-020-01431-z
- Xu X, Chemparathy A, Zeng L, et al. Engineered miniature CRISPR-Cas system for mammalian genome regulation and editing. Mol Cell. 2021;81(20). doi:10.1016/j.molcel.2021.08.008
- Phys Org. Researchers develop an engineered "mini" CRISPR genome editing system. Published September 3, 2021. Accessed December 22, 2022. phys.org/news/2021-09-mini-crispr-genome.html
- New Atlas. Protein tweak makes CRISPR gene editing 4,000 times less error-prone. Published March 3, 2022. Accessed December 22, 2022. newatlas.com/science/crispr-gene-editing-error-correction-protein/
- Bravo JPK, Liu M sen, Hibshman GN, et al. Structural basis for mismatch surveillance by CRISPR–Cas9. Nature. 2022;603(7900). doi:10.1038/s41586-022-04470-1
- Kato K, Zhou W, Okazaki S, et al. Structure and engineering of the type III-E CRISPR-Cas7-11 effector complex. Cell. 2022;185(13):2324-2337. doi:10.1016/j.cell.2022.05.003
- Roy S, Sanz Juste S, Sneider M, et al. Cas9/Nickase-Induced Allelic Conversion by Homologous Chromosome-Templated Repair in Drosophila Somatic Cells. Vol 8.; 2022. www.science.org
- New Scientist. 'Softer' form of CRISPR may edit genes more accurately. Published 2022. Accessed December 22, 2022. www.newscientist.com/article/2326976-softer-form-of-crispr-may-edit-genes-more-accurately/
- Anzalone, A.V., Koblan, L.W. & Liu, D.R. Genome editing with CRISPR–Cas nucleases, base editors, transposases and prime editors. Nat Biotechnol 38, 824–844 (2020). https://doi.org/10.1038/s41587-020-0561-9
- Puig-Serra, Pilar & Casado-Rosas, Maria & Martínez-Lage, Marta & Olalla-Sastre, Beatriz & Alonso-Yanez, Alejandro & Torres Ruiz, Raul & Rodríguez-Perales, Sandra. (2022). CRISPR Approaches for the Diagnosis of Human Diseases. International Journal of Molecular Sciences. 23. 1757. 10.3390/ijms23031757.