Put simply, electrophysiology is the study of the electrical properties of living cells and tissues. In practice, it involves investigating how specialized proteins known as ion channels regulate the flow of ions across cell membranes to effect various functions. This article explores the basics of electrophysiology and looks at some of the different techniques being used.
Membrane potential
Every cell has a membrane potential, which is defined as the electrical potential difference (or voltage) between the interior and the exterior. This results from the uneven distribution of ions (predominantly Na+, K+, Ca2+ and Cl-) on either side of the plasma membrane and underpins a broad range of cellular processes, including cell-volume control, proliferation, and wound healing1. While most cells maintain a membrane potential of around -70 mV, meaning that the inside of the cell is more negative than the outside, excitable cell types (neurons and muscle cells) are able to switch from their resting state and generate an action potential. In neurons, action potentials allow for communicating with other cells via the release of various neurotransmitters, while, in muscle cells, action potentials result in contraction.
Action potential
An action potential is a rapid change in membrane potential that is driven by voltage-gated ion channels in the plasma membrane. In neurons, it begins with the opening of voltage-gated Na+ channels, which causes an influx of Na+ ions that increases the membrane potential (depolarization). Next, the opening of voltage-gated K+ ion channels allows K+ ions to leave the cell, bringing the membrane potential back down towards its resting value (repolarization). Because the voltage-gated K+ ion channels are slightly delayed in closing, a short period of hyperpolarization occurs before the resting membrane potential is re-established. Following this process, the ions which were exchanged across the membrane are returned (albeit more slowly) by Na+/K+ ion pumps. The different phases of the neuronal action potential are shown in Figure 1. It should be noted that the duration and waveform of the cardiac action potential are different.
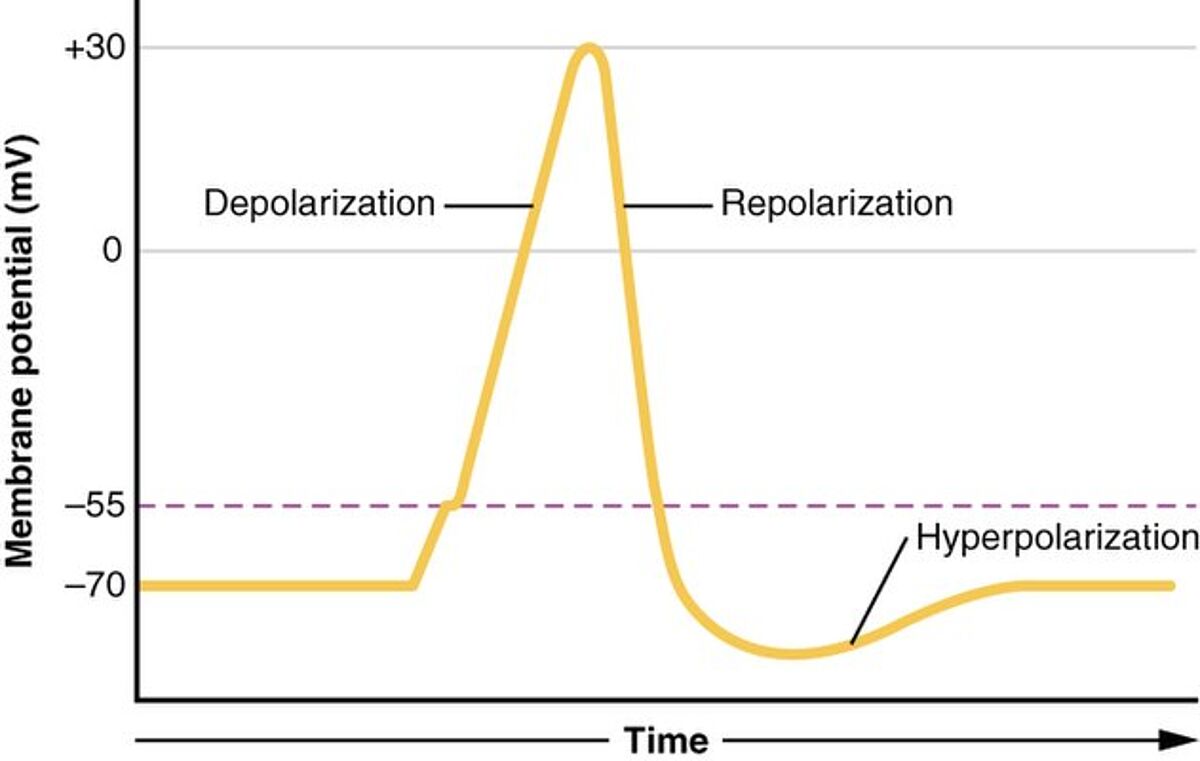
Electrophysiological techniques
Electrophysiological techniques can be broadly divided into two main categories based on whether they provide an intracellular or extracellular measurement. Patch-clamp electrophysiology, first reported by Erwin Neher and Bert Sakmann in the 1970s, is the best known example of the former, and led to the authors being awarded The Nobel Prize in Physiology or Medicine in 19912.
During patch-clamp electrophysiology, a glass or quartz micro-pipet containing an ionic solution is sealed onto the plasma membrane. This allows for any ions flowing through the membrane patch to enter the pipet, where they are recorded by a silver/silver-chloride wire electrode connected to an amplifier and converted to a digital signal. Since the development of the original method, other patch-clamp electrophysiology techniques have been described, including those for recording and manipulating currents flowing through single channels or across the entire cell membrane3.
Methods for extracellular recording involve layering cells or tissue sections over one or more electrodes in vitro or directly inserting probes into a living organism. A common approach when performing in vitro research is to use multielectrode arrays (MEAs), which offer significantly higher throughput compared to using just a single electrode. Importantly, MEAs enable researchers to investigate intercellular networking, which can reveal novel insights into the underlying mechanisms of both normal biology and disease.
Applications
Since many ion channels are linked to human pathologies, electrophysiology has broad utility, spanning everything from early-stage drug discovery and pre-clinical research through to clinical diagnosis and patient monitoring. For example, in the drug discovery setting, patch-clamp electrophysiology has long been used to identify and characterize inhibitors of the Nav1.7 ion channel, one of the best validated therapeutic targets for chronic pain4. And, for pre-clinical research, in vivo whole cell patch-clamp recordings from anaesthetized animals have allowed for investigating the neocortex, hippocampus, and other brain regions5. Within the realm of clinical electrophysiology, established practices include electrocardiography (ECG), electroencephalography (EEG), and electromyography (EMG), which are respectively used for measuring heart, brain, and muscle function, in addition to both auditory and visual testing.
Supporting your research
LubioScience represents some of the most trusted brands in research and works closely with our partners to offer an extensive selection of high-quality products for electrophysiology. Contact us today to discuss how we can support your project.
Supplier

AAT Bioquest
AAT Bioquest's reliable, high-performance products enable life science researchers to better understand biochemistry, immunology, cell biology and molecular biology.
Discover AAT Bioquest’s range of calcium dyes here.
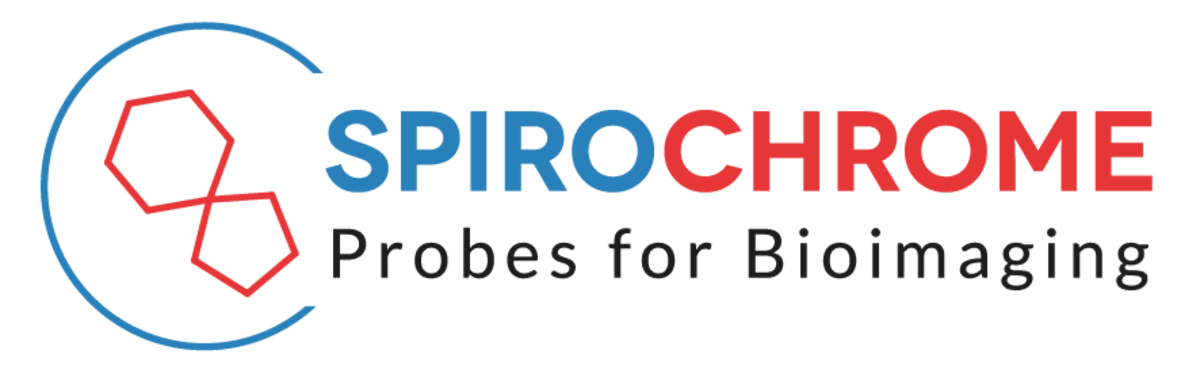
Spirochrome - live imaging probes
Spirochrome offers a broad range of fluorescent probes for live cell bioimaging. These include actin, tubulin, DNA membrane tension, cytoskeleton and lysosome probes.
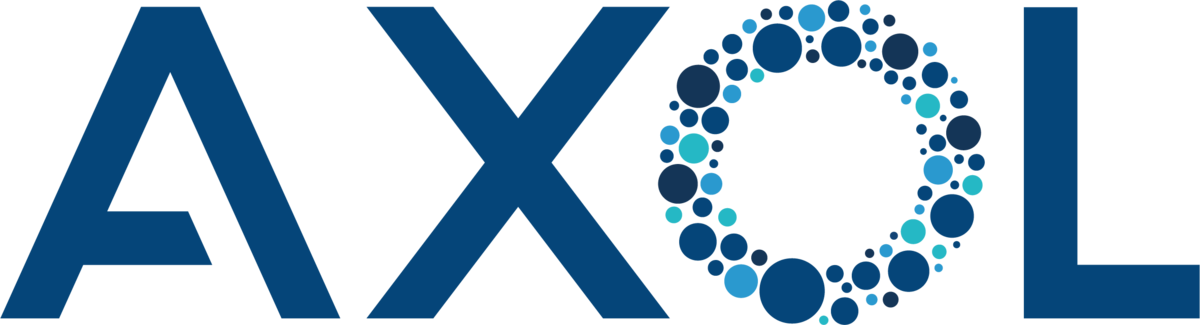
Axol Bioscience
Axol Bioscience offers high-quality, functionally relevant human derived iPSC-derived cells. This UK-based supplier has over 10 years of history producing tools for in vitro research and disease modeling for projects in neuroscience, pain/sensation and cardiovascular research.
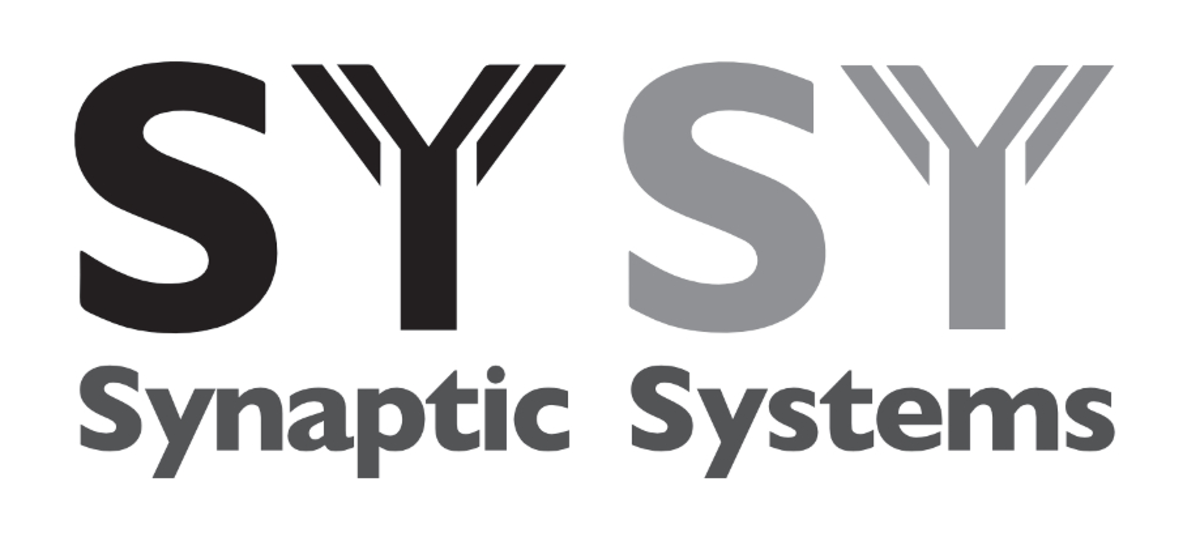
Synaptic systems
Antibodies for neuroscience & cell biology, camelid single domain FluoTags, HistoSure antibodies for histopathology, secondary antibodies, kits & lysates.
More about Synaptic Systems Shop for Synaptic Systems products
Discover our range of inhibitors and activators.
Explore our range of cation antibodies.